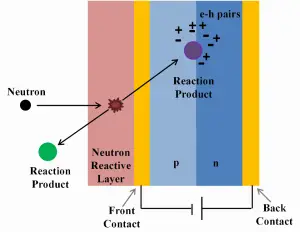
Source: large.stanford.edu
Neutron dosimetry is very specific, since the neutrons are electrically neutral particles, thus they are mainly subject to strong nuclear forces but not to electric forces. Therefore neutrons are not directly ionizing and they have usually to be converted into charged particles before they can be detected. Generally every type of neutron detector must be equipped with converter (to convert neutron radiation to common detectable radiation) and one of the conventional radiation detectors (scintillation detector, gaseous detector, semiconductor detector, etc.).
Studies have shown that alpha and neutron radiation cause greater biological damage for a given energy deposition per kg of tissue than gamma radiation does. It was discovered, biological effects of any radiation increases with the linear energy transfer (LET). In short, the biological damage from high-LET radiation (alpha particles, protons or neutrons) is much greater than that from low-LET radiation (gamma rays). This is because the living tissue can more easily repair damage from radiation that is spread over a large area than that which is concentrated in a small area. Because more biological damage is caused for the same physical dose (i.e., the same energy deposited per unit mass of tissue), one gray of alpha or neutron radiation is more harmful than one gray of gamma radiation. This fact that radiations of different types (and energies) give different biological effects for the same absorbed dose is described in terms of factors known as the relative biological effectiveness (RBE) and the radiation weighting factor (wR).
Radiation Weighting Factors – ICRP
For photon and electron radiation, the radiation weighting factor has the value 1 independently of the energy of the radiation and for alpha radiation the value 20. For neutron radiation, the value is energy-dependent and amounts to 5 to 20.
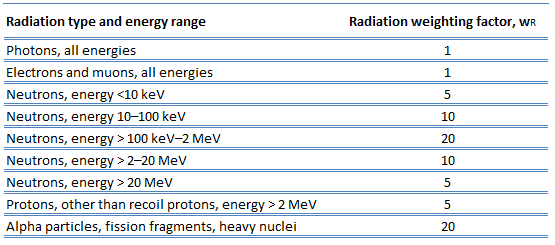
In 2007 ICRP published a new set of radiation weighting factors(ICRP Publ. 103: The 2007 Recommendations of the International Commission on Radiological Protection). These factors are given below.
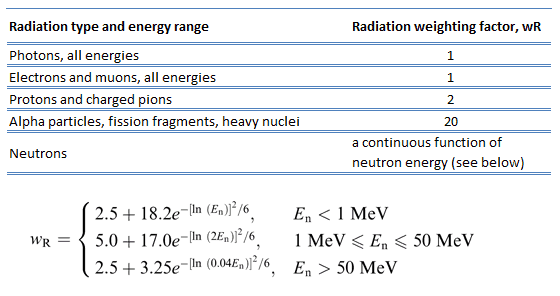
As shown in the table, a wR of 1 is for all low-LET radiations, i.e. X-rays and gamma rays of all energies as well as electrons and muons. A smooth curve, considered an approximation, was fitted to the wRvalues as a function of incident neutron energy. Note that En is the neutron energy in MeV.
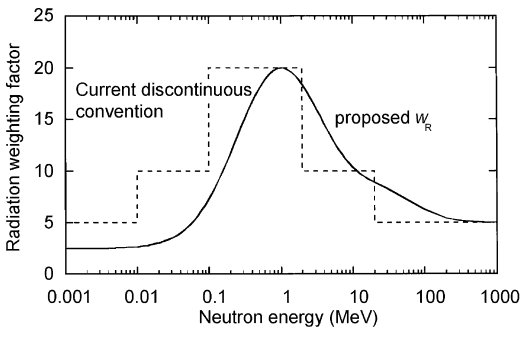
Thus for example, an absorbed dose of 1 Gy by alpha particles will lead to an equivalent dose of 20 Sv, and an equivalent dose of radiation is estimated to have the same biological effect as an equal amount of absorbed dose of gamma rays, which is given a weighting factor of 1.
Detection of Thermal Neutrons
Thermal neutrons are neutrons in thermal equilibrium with a surrounding medium of temperature 290K (17 °C or 62 °F). Most probable energy at 17°C (62°F) for Maxwellian distribution is 0.025 eV (~2 km/s). This part of neutron’s energy spectrum constitutes most important part of spectrum in thermal reactors.
Thermal neutrons have a different and often much larger effective neutron absorption cross-section (fission or radiative capture) for a given nuclide than fast neutrons.
In general, there are many detection principles and many types of detectors. In nuclear reactors, gaseous ionization detectors are the most common, since they are very efficient, reliable and cover a wide range of neutron flux. Various types of gaseous ionization detectors constitute so called the excore nuclear instrumentation system (NIS). The excore nuclear instrumentation system monitors the power level of the reactor by detecting neutron leakage from the reactor core.
Detection of Neutrons using Ionization Chamber
Ionization chambers are often used as the charged particle detection device. For example, if the inner surface of the ionization chamber is coated with a thin coat of boron, the (n,alpha) reaction can take place. Most of (n,alpha) reactions of thermal neutrons are 10B(n,alpha)7Li reactions accompanied by 0.48 MeV
Moreover, isotope boron-10 has high (n,alpha) reaction cross-section along the entire neutron energy spectrum. The alpha particle causes ionization within the chamber, and ejected electrons cause further secondary ionizations.
Another method for detecting neutrons using an ionization chamber is to use the gas boron trifluoride (BF3) instead of air in the chamber. The incoming neutrons produce alpha particles when they react with the boron atoms in the detector gas. Either method may be used to detect neutrons in nuclear reactor. It must be noted, BF3 counters are usually operated in the proportional region.
Detection of Fast Neutrons
Fast neutrons are neutrons of kinetic energy greater than 1 MeV (~15 000 km/s). In nuclear reactors, these neutrons are usually named fission neutrons. The fission neutrons have a Maxwell-Boltzmann distribution of energy with a mean energy (for 235U fission) 2 MeV. Inside a nuclear reactor the fast neutrons are slowed down to the thermal energies via a process called neutron moderation. These neutrons are also produced by nuclear processes such as nuclear fission or (ɑ,n) reactions.
In general, there are many detection principles and many types of detectors. Bu it must be added, detection of fast neutrons is very sophisticated discipline, since fast neutrons cross section are much smaller than in the energy range for slow neutrons. Fast neutrons are often detected by first moderating (slowing) them to thermal energies. However, during that process the information on the original energy of the neutron, its direction of travel, and the time of emission is lost.
Proton Recoil – Recoil Detectors
The most important type of detectors for fast neutrons are those which directly detect recoil particles, in particular recoil protons resulting from elastic (n, p) scattering. In fact, only hydrogen and helium nuclei are light enough for practical application. In the latter case the recoil particles are detected in a detector. Neutrons can transfer more energy to light nuclei. This method is appropriate for detecting fast neutrons allowing detection of fast neutrons without a moderator. This methods allows the energy of the neutron to be measured together with the neutron fluence, i.e. the detector can be used as a spectrometer. Typical fast neutron detectors are liquid scintillators, helium-4 based noble gas detectors and plastic detectors (scintillators). For example, the plastic has a high hydrogen content, therefore, it is useful for fast neutron detectors, when used as a scintillator.
Bonner Spheres Spectrometer
There are several methods for detecting slow neutrons, and few methods for detecting fast neutrons. Therefore, one technique for measuring fast neutrons is to convert them to slow
neutrons, and then measure the slow neutrons. One of possible methods is based on Bonner spheres. The method was first described in 1960 by Ewing and Tom W. Bonner and employs thermal neutron detectors (usually inorganic scintillators such as 6LiI) embedded in moderating spheres of different sizes. Bonner spheres have been used widely for the measurement of neutron spectra with neutron energies ranged from thermal up to at least 20 MeV. A Bonner sphere neutron spectrometer (BSS) consists of a thermal-neutron detector, a set polyethylene spherical shells and two optional lead shells of various sizes. In order to detect thermal neutrons a 3He detector or inorganic scintillators such as 6LiI can be used. LiGlass scintillators are very popular for detection of thermal neutrons. The advantage of LiGlass scintillators is their stability and their large range of sizes.
Detection of Neutrons using Scintillation Counter
Scintillation counters are used to measure radiation in a variety of applications including hand held radiation survey meters, personnel and environmental monitoring for radioactive contamination, medical imaging, radiometric assay, nuclear security and nuclear plant safety. They are widely used because they can be made inexpensively yet with good efficiency, and can measure both the intensity and the energy of incident radiation.
Scintillation counters can be used to detect alpha, beta, gamma radiation. They can be used also for detection of neutrons. For these purposes, different scintillators are used.
- Neutrons. Since the neutrons are electrically neutral particles, they are mainly subject to strong nuclear forces but not to electric forces. Therefore neutrons are not directly ionizing and they have usually to be converted into charged particles before they can be detected. Generally every type of neutron detector must be equipped with converter (to convert neutron radiation to common detectable radiation) and one of the conventional radiation detectors (scintillation detector, gaseous detector, semiconductor detector, etc.). Fast neutrons (>0.5 MeV) primarily rely on the recoil proton in (n,p) reactions. Materials rich in hydrogen, for example plastic scintillators, are therefore best suited for their detection. Thermal neutrons rely on nuclear reactions such as the (n,γ) or (n,α) reactions, to produce ionization. Materials such as LiI(Eu) or glass silicates are therefore particularly well-suited for the detection of thermal neutrons. The advantage of 6LiGlass scintillators is their stability and their large range of sizes.
Neutron Thermoluminescent Dosimeter – Neutron TLD
The personnel neutron dosimetry continues to be one of the problems in the field of radiation protection, as no single method provides the combination of energy response, sensitivity, orientation dependence characteristics and accuracy necessary to meet the needs of a personnel dosimeter.
The most commonly used personnel neutron dosimeters for radiation protection purposes are thermoluminescent dosimeters and albedo dosimeters. Both are based on this phenomenon – thermoluminescence. For this purpose, lithium fluoride (LiF) as sensitive material (chip) is widely used. Lithium fluoride TLD is used for gamma and neutron exposure (indirectly, using the Li-6 (n,alpha)) nuclear reaction. Small crystals of LiF (lithium fluoride) are the most common TLD dosimeters since they have the same absorption properties as soft tissue. Lithium has two stable isotopes, lithium-6 (7.4 %) and lithium-7 (92.6 %). Li-6 is the isotope sensitive to neutrons. In order to record neutrons, LiF crystal dosimeters may be enriched in lithium-6 to enhance the lithium-6 (n,alpha) nuclear reaction. The efficiency of the detector depends on the energy of the neutrons. Because the interaction of neutrons with any element is highly dependent on energy, making a dosimeter independent of the energy of neutrons is very difficult. In order to separate thermal neutrons and photons, LiF dosimeters are mostly utilized, containing different percentage of lithium-6. LiF chip enriched in lithium-6, which is very sensitive to thermal neutrons and LiF chip containing very little of lithium-6, which has a negligible neutron response.
The principle of neutron TLDs is then similar as for gamma radiation TLDs. In the LiF chip, there are impurities (e.g. manganese or magnesium), which produce trap states for energetic electrons. The impurity causes traps in the crystalline lattice where, following irradiation (to alpha radiation), electrons are held. When the crystal is warmed, the trapped electrons are released and light is emitted. The amount of light is related to the dose of radiation received by the crystal.
Thermoluminescent Albedo Neutron Dosimeter
Albedo neutron dosimetry is based on the effect of moderation and backscattering of neutrons by the human body. Albedo, the latin word for “whiteness”, was defined by Lambert as the fraction of the incident light reflected diffusely by a surface. Moderation and backscattering of neutrons by the human body creates a neutron flux at the body surface in the thermal and intermediate energy range. These backscattered neutrons called albedo neutrons, can be detected by a dosimeter (usually a LiF TLD chip), placed on the body which is designed to detect thermal neutrons. Albedo dosimeters have been found to be the only dosimeters which can measure doses due to neutrons over the whole range of energies. Usually, two types of lithium fluoride are used to separate doses contributed by gamma-rays and neutrons. LiF chip enriched in lithium-6, which is very sensitive to thermal neutrons and LiF chip containing very little of lithium-6, which has a negligible neutron response.
We hope, this article, Neutron Dosimetry – Neutron Dosimeter, helps you. If so, give us a like in the sidebar. Main purpose of this website is to help the public to learn some interesting and important information about radiation and dosimeters.