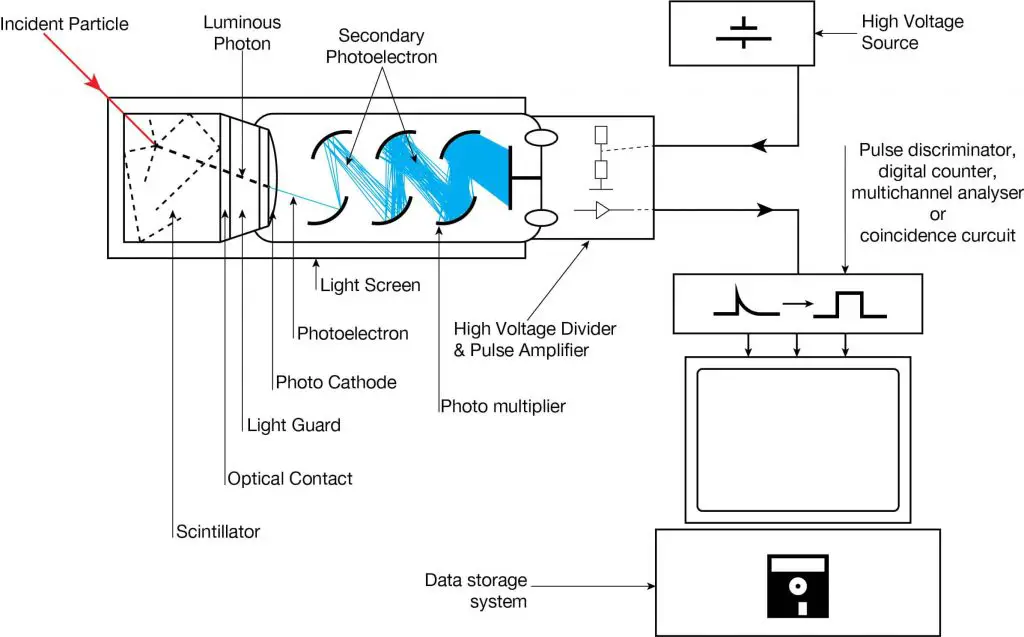
A scintillation counter or scintillation detector is a radiation detector which uses the effect known as scintillation. Scintillation is a flash of light produced in a transparent material by the passage of a particle (an electron, an alpha particle, an ion, or a high-energy photon). Scintillation occurs in the scintillator, which is a key part of a scintillation detector. In general, a scintillation detector consists of:
- Scintillator. A scintillator generates photons in response to incident radiation.
- Photodetector. A sensitive photodetector (usually a photomultiplier tube (PMT), a charge-coupled device (CCD) camera, or a photodiode), which converts the light to an electrical signal and electronics to process this signal.
Scintillation counters are widely used in radiation protection, assay of radioactive materials and physics research because they can be made inexpensively yet with good efficiency, and can measure both the intensity and the energy of incident radiation. Hospitals all over the world have gamma cameras based on the scintillation effect and, therefore, they are also called scintillation cameras.
The advantages of a scintillation counter are its efficiency and the high precision and counting rates that are possible. These latter attributes are a consequence of the extremely short duration of the light flashes, from about 10-9 (organic scintillators) to 10-6 (inorganic scintillators) seconds. The intensity of the flashes and the amplitude of the output voltage pulse are proportional to the energy of the radiation. Therefore, scintillation counters can be used to determine the energy, as well as the number, of the exciting particles (or gamma photons). For gamma spectrometry, the most common detectors include sodium iodide (NaI) scintillation counters and high-purity germanium detectors.
Applications of Scintillation Counters
Scintillation counters are used to measure radiation in a variety of applications including hand held radiation survey meters, personnel and environmental monitoring for radioactive contamination, medical imaging, radiometric assay, nuclear security and nuclear plant safety. They are widely used because they can be made inexpensively yet with good efficiency, and can measure both the intensity and the energy of incident radiation.
Scintillation counters can be used to detect alpha, beta, gamma radiation. They can be used also for detection of neutrons. For these purposes, different scintillators are used:
-
CsI(Tl) scintillation crystal. Source: wikipedia.de License: CC BY-SA 3.0 Alpha Particles and Heavy Ions. Due to the very high ionizing power of heavy ions, scintillation counters are usually not ideal for the detection of heavy ions. For equal energies, a proton will produce 1/4 to 1/2 the light of an electron, while alpha particles will produce only about 1/10 the light. Where needed, inorganic crystals, e.g. CsI(Tl), ZnS(Ag) (typically used in thin sheets as α-particle monitors), should be preferred to organic materials. Pure CsI is a fast and dense scintillating material with relatively low light yield that increases significantly with cooling. The drawbacks of CsI are a high temperature gradient and a slight hygroscopicity.
- Beta Particles. For detection of beta particles, organic scintillators can be used. Pure organic crystals include crystals of anthracene, stilbene and naphthalene. The decay time of this type of phosphor is approximately 10 nanoseconds. This type of crystal is frequently used in the detection of beta particles. Organic scintillators, having a lower Z than inorganic crystals, are best suited for the detection of low-energy (< 10 MeV) beta particles.
- Gamma Rays. High-Z materials are best suited as scintillators for the detection of gamma rays. The most widely used scintillation material is NaI(Tl) (thallium-doped sodium iodide). The iodine provides most of the stopping power in sodium iodide (since it has a high Z = 53). These crystalline scintillators are characterized by high density, high atomic number, and pulse decay times of approximately 1 microsecond (~ 10-6 sec). Scintillation in inorganic crystals is typically slower than in organic ones. They exhibit high efficiency for detection of gamma rays and are capable of handling high count rates. Inorganic crystals can be cut to small sizes and arranged in an array configuration so as to provide position sensitivity. This feature is widely used in medical imaging to detect X-rays or gamma rays. Inorganic scintillators are better at detecting gamma rays and X-rays. This is due to their high density and atomic number which gives a high electron density.
- Neutrons. Since the neutrons are electrically neutral particles, they are mainly subject to strong nuclear forces but not to electric forces. Therefore neutrons are not directly ionizing and they have usually to be converted into charged particles before they can be detected. Generally every type of neutron detector must be equipped with converter (to convert neutron radiation to common detectable radiation) and one of the conventional radiation detectors (scintillation detector, gaseous detector, semiconductor detector, etc.). Fast neutrons (>0.5 MeV) primarily rely on the recoil proton in (n,p) reactions. Materials rich in hydrogen, for example plastic scintillators, are therefore best suited for their detection. Thermal neutrons rely on nuclear reactions such as the (n,γ) or (n,α) reactions, to produce ionization. Materials such as LiI(Eu) or glass silicates are therefore particularly well-suited for the detection of thermal neutrons.
We hope, this article, Application of Scintillation Counters, helps you. If so, give us a like in the sidebar. Main purpose of this website is to help the public to learn some interesting and important information about radiation and dosimeters.