Alpha decay (or α-decay and also alpha radioactivity) represents the disintegration of a parent nucleus to a daughter through the emission of the nucleus of a helium atom. This transition can be characterized as:
As can be seen from the figure, alpha particle is emitted in alpha decay. Alpha particles are energetic nuclei of helium. Alpha particles consist of two protons and two neutrons bound together into a particle identical to a helium nucleus. Alpha particles are relatively large and carry a double positive charge. They are not very penetrating and a piece of paper can stop them. They travel only a few centimeters but deposit all their energies along their short paths.
In practice, this mode of decay has only been observed in nuclides considerably heavier than nickel, with the lightest known alpha emitters being the lightest isotopes (mass numbers 106–110) of tellurium (element 52). In nuclear reactors alpha decay occurs for example in the fuel (alpha decay of heavy nuclei). Alpha particles are commonly emitted by all of the heavy radioactive nuclei occuring in the nature (uranium, thorium or radium), as well as the transuranic elements (neptunium, plutonium or americium).
Theory of Alpha Decay – Quantum Tunneling
Among the variety of channels in which a nucleus decays, alpha decay has been one of the most studied. The alpha decay channel in heavy and super heavy nuclei has provided information on the fundamental properties of nuclei far from stability, such as their ground state energies and the structure of their nuclear levels.
Alpha decay is a quantum tunneling process. In order to be emitted, the alpha particle must penetrate a potential barrier. This is similar to cluster decay, in which an atomic nucleus emits a small “cluster” of neutrons and protons (e.g. 12C).
The height of the Coulomb barrier for nuclei of A « 200 is about 20-25 MeV. The alpha particles emitted in nuclear decay have typical energies of about 5 MeV. On the one hand an incoming 5 MeV alpha particle is scattered from a heavy nucleus and it cannot penetrate the Coulomb barrier and get sufficiently close to the nucleus to interact via the strong force. On the other hand, a 5 MeV alpha particle bound in a nuclear potential well is able to tunnel that same Coulomb barrier.
By 1928, George Gamow (and independently by Ronald Gurney and Edward Condon) had solved the theory of alpha decay via quantum tunneling. They assumed that the alpha particle and the daughter nucleus exist within the parent nucleus prior to its dissociation, namely the decay of quasistationary states (QS). A quasistationary state is defined as a long-lived state that eventually decays. Initially, the alpha cluster oscillates in the potential of the daughter nucleus, with the Coulomb potential preventing their separation. The alpha particle is trapped in a potential well by the nucleus. Classically, it is forbidden to escape, but according to the (then) newly discovered principles of quantum mechanics, it has a tiny (but non-zero) probability of “tunneling” through the barrier and appearing on the other side to escape the nucleus. Using the tunneling mechanism, Gamow, Condon and Gurney calculated the penetrability of the tunneling α particle through the Coulomb barrier, finding the lifetimes of some α emitting nuclei. The main success of this model was the reproduction of the semi-empirical Geiger-Nuttall law that expresses the lifetimes of the α emitters in terms of the energies of the released α particles. It must be noted, that other common forms of decay (e.g. beta decay) are governed by the interplay between both the nuclear force and the electromagnetic force.
Special Reference: W.S.C. Williams. Nuclear and Particle Physics. Clarendon Press; 1 edition, 1991, ISBN: 978-0198520467.
Gamma decay or γ decay represents the disintegration of a parent nucleus to a daughter through the emission of gamma rays (high energy photons). This transition (γ decay) can be characterized as:
As can be seen, if a nucleus emits a gamma ray, atomic and mass numbers of daughter nucleus remain the same, but daughter nucleus will form different energy state of the same element. Note that, nuclides with equal proton number and equal mass number (thus making them by definition the same isotope), but in a different energy state are known as nuclear isomers. We usually indicate isomers with a superscript m, thus: 241mAm or 110mAg.
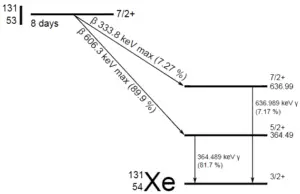
In most practical laboratory sources, the excited nuclear states are created in the decay of a parent radionuclide, therefore a gamma decay typically accompanies other forms of decay, such as alpha or beta decay. Typically after a beta decay (isobaric transition), nuclei usually contain too much energy to be in its final stable or daughter state.
Gamma rays are high-energy photons with very short wavelengths and thus very high frequency. Gamma rays from radioactive decay are in the energy range from a few keV to ~8 MeV, corresponding to the typical energy levels in nuclei with reasonably long lifetimes. As was written, they are produced by the decay of nuclei as they transition from a high energy state to a lower state. Since the gamma rays are in substance only a very high-energy photons, they are very penetrating matter and are thus biologically hazardous. Gamma rays can travel thousands of feet in air and can easily pass through the human body.
In contrast to alpha and beta radioactivity, gamma radioactivity is governed by an electromagnetic interaction rather than a weak or strong interaction. As in atomic transitions, the photon carries away at least one unit of angular momentum (the photon, being described by the vector electromagnetic field, has spin angular momentum of ħ), and the process conserves parity.
Special Reference: W.S.C. Williams. Nuclear and Particle Physics. Clarendon Press; 1 edition, 1991, ISBN: 978-0198520467.
We hope, this article, Alpha Decay vs Gamma Decay – Radioactivity, helps you. If so, give us a like in the sidebar. Main purpose of this website is to help the public to learn some interesting and important information about radiation and dosimeters.