Description of Beta Particles
Beta particles are high-energy, high-speed electrons or positrons emitted by certain fission fragments or by certain primordial radioactive nuclei such as potassium-40. The beta particles are a form of ionizing radiation also known as beta rays. The production of beta particles is termed beta decay. There are two forms of beta decay, the electron decay (β− decay) and the positron decay (β+ decay). In a nuclear reactor occurs especially the β− decay, because the common feature of the fission products is an excess of neutrons (see Nuclear Stability). An unstable fission fragment with the excess of neutrons undergoes β− decay, where the neutron is converted into a proton, an electron, and an electron antineutrino.
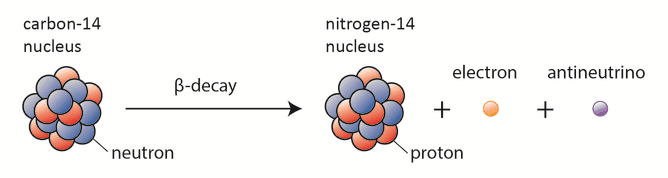
Characteristics of Beta Radiation
Key characteristics of beta radiation are summarized in following points:
- Beta particles are energetic electrons, they are relatively light and carry a single negative charge.
- Their mass is equal to the mass of the orbital electrons with which they are interacting and unlike the alpha particle a much larger fraction of its kinetic energy can be lost in a single interaction.
- Their path is not so straightforward. The beta particles follow a very zig-zag path through absorbing material. This resulting path of particle is longer than the linear penetration (range) into the material.
- Since they have very low mass, beta particles reach mostly relativistic energies.
- Beta particles also differ from other heavy charged particles in the fraction of energy lost by radiative process known as the bremsstrahlung. Therefore for high energy beta radiation shielding dense materials are inappropriate.
- When the beta particle moves faster than the speed of light (phase velocity) in the material it generates a shock wave of electromagnetic radiation known as the Cherenkov radiation.
- The beta emission has the continuous spectrum.
- A 1 MeV beta particle can travel approximately 3.5 meters in air.
- Due to the presence of the bremsstrahlung low atomic number (Z) materials are appropriate as beta particle shields.
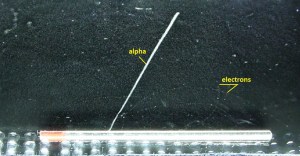
Source: wikipedia.org
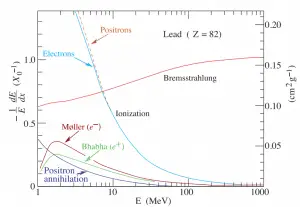
function of electron or positron energy. Source: http://pdg.lbl.gov/
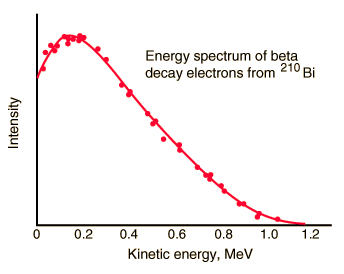
We hope, this article, Characteristics of Beta Radiation / Particles, helps you. If so, give us a like in the sidebar. Main purpose of this website is to help the public to learn some interesting and important information about radiation and dosimeters.